Biospheric Theory and Modelling
1. Overview
Energy conversions between different forms is at the center of life, human societies and the entire earth system. When sunlight reaches the surface of the earth, its energy can simply raise the temperature and then it is converted into thermal energy. It can create buoyancy in the atmosphere that creates kinetic energy, or it can evaporate water, a form of latent heat. Sunlight can also be used by vegetation for photosynthesis, where it is converted into chemical energy in the form of carbohydrates. Or it hits a solar panel and generates electricity for human consumption.
There are strict physical laws that regulate the conversion of energy, the so-called laws of thermodynamics. One of these laws is the conservation of energy. Whenever energy changes shape, nothing is lost or gained overall. The other relevant law deals with entropy, which gives the direction and relevant limits for energy conversions. Nevertheless, it is practically not taken into account in current Earth system research.
Our group takes thermodynamics as a starting point, but combines it with the interactions within the earth system. From this we deduce how the system should work. One example is atmospheric circulation, the fundamental transport mechanism that enables global biogeochemical cycles. The kinetic energy of the atmosphere is created by differences in heating and cooling, and the laws of thermodynamics limit the rate of conversion. As it turns out, the atmosphere works as hard as possible to create movement. The conversion of energy and the laws of thermodynamics thus represent an extremely important limitation of the atmospheric circulation.
This has several implications: It suggests that other processes in the Earth system are also working as hard as they can. This makes them highly predictable through such thermodynamic approaches. It offers a simple approach, deeply ingrained in basic physics, to describe the role of motion. So we can make simple, physical estimates of the climate and its changes. It may not be as precise as a complex computer model, but it does provide insight into the most important factors. And this approach has practical implications: it determines how much renewable energy the earth will produce at best. Our group develops this approach further and applies it to other topics in Earth system research.
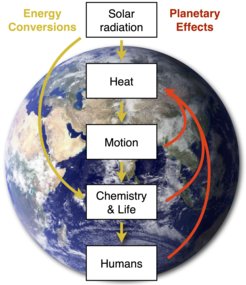
2. Earth system theory
We explore how the thermodynamic Earth system approach can be applied to different fields and questions in Earth system science. Some research is quite theoretical, e.g., aiming to link the intensity of energy conversion with characteristic abundance distributions or the spatial organization of systems. Other exploratory work aims to fully describe hydrologic cycling as a thermodynamic system to infer its basic characteristics and how these should change with climate change. We also investigate the application of these concepts to the dynamics of ecosystems, to the factors that allow for life on a planet, and to the potential dynamics of advanced civilizations on exoplanets.
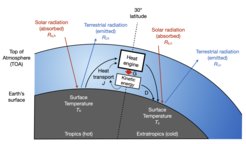
Review article (in German)
Kleidon, A. (2012). Was leistet die Erde? Physik in unserer Zeit. 43(3), 136-144.
doi: 10.1002/piuz.201201294.
3. Land-atmosphere interactions
We apply our approach to understand the basic factors that shape climate on land and test these estimates with observations. One focus is on understanding how the incoming solar energy is partitioned into different energy forms during the day. This partitioning is mostly shaped by thermodynamics. We then evaluate how vegetation, water availability and the atmosphere alter this partitioning. Vegetation develops deep root systems that alter the access to soil water. This changes water availability, energy partitioning, and how the atmosphere buffers diurnal variations.
This approach is applied to understand the variations in climate and water cycling across regions. We also apply our approach to learn how these dynamics change with global warming.
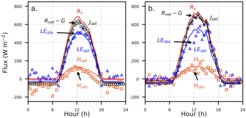
Overview article (in German)
Kleidon, A., Renner, M. (2015). Geoengineering ist keine Lösung -- Der globale Wasserkreislauf im Klimasystem. Physik in unserer Zeit, 46(1), 27-31.
doi: 10.1002/piuz.201401381.
Kleidon, A. (2020). Sonne oder Treibhauseffekt? Globale Erwärmung einfach und physikalisch nachgerechnet. Physik in unserer Zeit, 51(2), 79-85.
doi: 10.1002/piuz.202001560.
4. Renewable energy
The energy conversions of the whole Earth system set the magnitudes of different forms of renewable energy. When more conversion steps are involved, his results in a lower resource potential. Hence, using solar radiation directly, for instance by photovoltaics, has by far the largest resource otential, even in the not-so-sunny Germany. Wind energy involves more steps and has a lower resource potential. It becomes even less the more wind turbines take kinetic energy out of the atmosphere. Photosynthesis has a very low efficiency of converting sunlight into chemical energy. This results in a low resource potential for different forms of biofuels. We develop simplified models to infer these magnitudes and evaluate scenarios for the transition to a sustainable energy system.
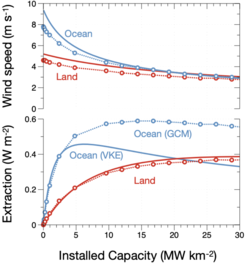
the wind resource potential (lower plot). These insights can be inferred from energetic principles (lines). Kleidon (2021) doi: 10.1127/metz/2021/1062.
Overview article (in German)
Kleidon, A. (2019). Sonne statt Flaute. Physik in unserer Zeit, 50(3), 120-127.
doi: 10.1002/piuz.201901540.